From the plagues of medieval Europe to the influenza pandemic of 1918, the specter of the next public health disaster has gripped the minds of scientists, captivated the imaginations of writers and vexed conspiracy theorists.
Now, a new coronavirus is engulfing the world, and the long-foretold once-in-a-century pandemic has become a reality.
New insights are accumulating rapidly, but many things about the virus, SARS-CoV-2, and the disease that it causes, COVID-19, remain unknown. One thing, however, is certain, experts say: This pandemic will not be the last.
The newly formed Massachusetts Consortium on Pathogen Readiness aims to address both the immediate and long-term implications of the crisis. The effort, led by Harvard Medical School, will work to stem the tide of COVID-19 but, more importantly, to lay the groundwork for dealing with future pandemics.
“This is a historic moment. It reminds us why we have dedicated our lives and careers to science and medicine,” said HMS Dean George Q. Daley. “The novel coronavirus is a public health crisis of cataclysmic proportions. It compels us to forge new levels of collaboration across Boston, Cambridge and beyond to address both the immediate challenges of this pandemic and to create a rapid-response system to deal with future crises.”
The initiative, formalized at a March 2 meeting at HMS, was sparked by a collaborative research grant from the China Evergrande Group. Under the research agreement, $115 million will be shared equally between Boston-based researchers and colleagues at the Guangzhou Institute of Respiratory Health.
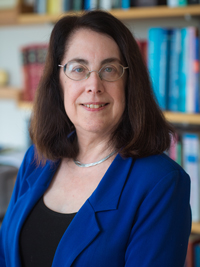
The Boston-based consortium, spearheaded by Daley and faculty co-leads Arlene Sharpe and Bruce Walker, already involves more than 100 scientists across five area universities and institutes, as well as Harvard-affiliated hospitals. More scientists are joining the ranks daily.
“This is an extraordinary, collaborative effort which brings together scientists and clinicians from across Boston’s research community to tackle this crisis and prepare for potential future outbreaks,” said Sharpe, chair of the Department of Immunology in the Blavatnik Institute at HMS. "The response to COVID-19 is unprecedented and truly inspiring."
Walker, a virologist and HIV expert, describes the new coronavirus as an existential threat that demands pan-institutional and transnational alliances.
“This is not business as usual,” said Walker, the Phillip T. and Susan M. Ragon Professor of Medicine at Massachusetts General Hospital and professor of immunology at HMS. “There’s a huge amount of knowledge that can be applied collectively and collaboratively. There is a phenomenal sense of energy and commitment from everyone involved, and the important thing is that we sustain it. We are now moving beyond institutional allegiances and allying with each other to try to solve this critical problem.”
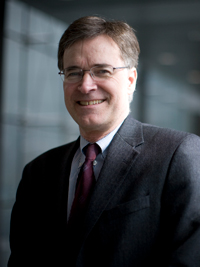
Evidence about the new virus—its biology, its behavior, its effects on the body—is growing by the day, with papers posted on preprint servers for the global scientific community to dissect and pull from before the research has undergone standard peer review. Across the globe, there are multiple vaccine trials and a growing number of clinical trials testing experimental treatments for COVID-19.
As scientists forge ahead to piece together a comprehensive profile of the virus fueling this historic pandemic, researchers are focusing their efforts on six areas: epidemiology, diagnostics, pathogenesis, clinical disease management, treatment and vaccines.
EPIDEMIOLOGY
During pandemics, epidemiologists work as profilers. They trace, monitor and predict the movement and behavior of a pathogen in an effort to create a detailed portrait of an invisible foe that inflicts damage that is all too real. Key epidemiological questions include: What is the spectrum of disease severity? How transmissible is the virus and who is most likely to spread it? What are the risk factors for severe illness and death? How does the behavior of the infection vary by location, and does it change over time? Answers to these questions can inform key decisions about what public health measures should be implemented and what resources should be allocated—choices that can profoundly reshape outcomes. Moreover, epidemiologic trends can provide telltale clues for more focused areas of study. For example, if a certain group appears to be at high risk for severe complications (elderly people with cardiovascular illness or cancer) or seemingly resistant to disease (children), then clinical researchers and basic scientists could try to untangle the biologic factors and physiology of infection that underlie these observations.
To glean answers, epidemiologists rely on two tools: sophisticated disease modeling and old-fashioned raw data collection.
Megan Murray and Michael Mina, who co-lead the epidemiology working group of the new consortium, say that estimating the true spread of the virus is critical to answer both immediate and long-term questions about COVID-19. Understanding the true spread would not only reveal critical clues about the movement and transmissibility across different communities, but can also inform anticipatory decision-making.
Murray, professor of Global Health and Social Medicine in the Blavatnik Institute at HMS, is director of research for Partners in Health, an organization that helps create sustainable medical care and delivery in 10 nations with severely under-resourced health care systems. One of Murray’s recent projects is developing models to predict the movement of the new coronavirus across each of those nations and forecast the impact of the pandemic based on country-specific dynamics. These analyses can inform precision-targeted allocation of materials and human resources based on each region’s anticipated need.
Murray, working with Marc Lipsitch, professor of epidemiology, immunology and infectious diseases at the Harvard T.H. Chan School of Public Health, has also developed a model that predicts how many intensive-care beds would be needed in different parts of the United States. In partnership with a group in Malawi, Murray is modifying the model to estimate the number of intensive-care beds and hospital beds that will be needed in that country.
Lipsitch, who directs the Center for Communicable Disease Dynamics at the Harvard Chan School, underscores the value of granular raw data to inform best practices. To achieve predictive accuracy in computational modeling, he said, it is critical to gather surveillance data on both the macro and micro levels—at country, state, province, town, neighborhood, workplace and even household levels.
The ability to gather large amounts of data rapidly has made disease modeling increasingly accurate and reliable. Raw data combined with sophisticated modeling can yield insights such as whether social distancing regulations are being followed and whether they are making a dent in the epidemic.
So are current measures changing the course of the disease?
“We need sustained interventions like social distancing for long enough to ensure we’re going to see a decline in cases,” Murray said. “Short of that, we’re not going to see it go away by the miracle of spring. If I were the government, I would take that very seriously.”
Murray’s best prediction for the trajectory of the pandemic is that we’re in it for the long haul.
“Is there a magic drug that will suddenly fix everything? I doubt that there will be an overnight cure,” Murray said. “We’ll make steady incremental progress, but we’re going to do it much faster than in the past.”
Lipsitch says he is concerned that containment measures in the United States have been patchy and social distancing has not been practiced universally. He predicts that even if all necessary measures are implemented, there may be no true end in sight any time soon. The best outcome, he added, would be to have one bad wave of infections, contained by social distancing, followed by a level of controllable spread.
“There will be a world of COVID-19 transmission throwing sparks back at us,” Lipsitch wrote in a recent tweet. “Like China today, we will be in a long-term effort to prevent these sparks from starting new chains of transmission.”
DIAGNOSTICS
Testing for the novel coronavirus had a halting start in the United States, mostly due to an initial test from the Centers for Disease Control and Prevention that contained a problematic reagent leading to faulty readings. Since then, new, more accurate assays have been deployed, and testing has become more widely available. Yet, large-scale testing remains woefully behind, particularly in some areas of the country, and has yet to ramp up to needed levels. And new testing modalities are sorely needed, including:
- Ultra-sensitive assays that capture very low levels of viral proteins and enable rapid point-of-care testing
- Tests that detect biomarkers that herald the onset of an aberrant immune response, which has been linked to the development of critical complications
- Antibody-based testing that identifies individuals who have recovered from infections with the new coronavirus and have developed immunity to the pathogen
Some of these next-generation platforms are already in development.
Ultrasensitive point-of-care tests: Bioengineer David Walt, who co-leads the diagnostics arm of the consortium together with computational geneticist Pardis Sabeti, is exploring three different approaches.
Walt’s labs, at the Wyss Institute for Biologically Inspired Engineering and Brigham and Women’s Hospital, have already developed an ultra-sensitive method to detect proteins with up to a 1,000 times greater sensitivity than conventional methods. Now, Walt and team are adapting this method (Single Molecule Arrays, or SiMoA), to design an assay that detects SARS-CoV-2 proteins at ultralow levels, including protein fragments that have splintered from the virus. Such a test could detect minimal viral presence not only in standard throat swabs but also in saliva, which is not currently possible. Besides vastly enhancing the detection capacity of current tests, this method would also allow clinicians to perform rapid, point-of-care testing while the patient is in the clinic or in the physician’s office, yielding results in as little as 30 minutes, said Walt, the Hansjörg Wyss Professor of Biologically Inspired Engineering and of pathology at HMS and Brigham and Women’s. Current turnaround time on test results varies, but it can take anywhere from a few hours to a few days.
Critical disease biomarkers: Walt and colleague Galit Alter, HMS professor of medicine at Mass General, are working with samples from patients who developed acute respiratory distress syndrome, one of the hallmarks of severe COVID-19. The condition often requires mechanically assisted breathing in intensive care.
A critical area of uncertainty remains whether this complication is fueled by an exuberant immune system response that damages the lung tissue or whether the lung damage is inflicted directly by the virus itself. Could it be both? This uncertainty raises another question: If immune over-reactivity is at play, are certain people more prone to developing an exuberant immune reaction known as a cytokine storm, the widespread release of immune-signaling proteins that can cause cellular demise, tissue injury and organ damage? And if some people are more likely to develop such critical complications, are there early indicators that might herald the impending immune vortex? If such chemical biomarkers can be detected early enough, could this be a clue for physicians to treat preemptively patients on the brink of cytokine storms? Walt’s team is working on a new generation of tests to detect, early on, even the subtlest of upticks in levels of inflammatory molecules that portend cytokine storm. In theory, Walt said, this early detection would allow patients who are on the cusp of such life-threatening complications to be treated early and more aggressively to stave off the worst complications.
Antibody tests would be invaluable, experts agree, in several ways. They would identify people who have been infected with SARS-CoV-2—either with symptoms or silently—and have built up immunity against the virus. This knowledge could provide more realistic estimates of the virus’s spread, yield more accurate calculations of fatality rate and help inform vaccine development and treatments. Such work is already starting in the lab of HMS geneticist Stephen Elledge, the Gregor Mendel Professor of Genetics and of Medicine at HMS and Brigham and Women’s, who is adapting his VirScan tool to study antibody response following coronavirus infections.
Walt’s lab is developing ultrasensitive tests to detect seroconversion—the process during which an infected person's immune system produces neutralizing antibodies against the virus. Such a test could be used to identify people who have mounted an immune response to the virus and may be safe to return to work and a normal lifestyle, Walt said.
Antibody-based testing would also allow a more accurate understanding of how the immune system behaves after infection and how a person’s natural immunity might wane over time. Duane Wesemann, HMS associate professor of medicine and an immunologist at Brigham and Women’s, recently launched a study to explore this question. The research will compare immune responses in health care workers and the general public to determine whether the presence of symptoms during initial infection may be related to how robust the body’s immune response is. The study will also estimate the number of people who develop antibodies without symptoms and will isolate and characterize the most protective antibodies in an effort to inform reverse-engineering approaches to vaccine development.
To avoid testing delays, as well as other challenges, in future pandemics, experts say a better centralized response, both domestically and globally, is critical.
“The scientific, regulatory and public health communities have learned a lot and are continuing to learn a lot from this crisis,” Walt said. “While the tools individually work extremely well, frankly, we could do a lot better if we had a concerted collaborative mobilized effort that was positioned to anticipate and respond to these kinds of things.”
PATHOGENESIS: Understanding the disease, from protein to person
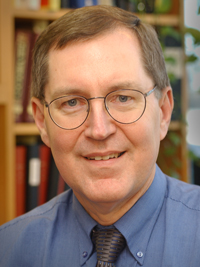
One of the central questions about the new coronavirus and the disease that it causes revolves around the host-pathogen interaction: What type of immune response does the virus induce inside infected cells, tissues and organs?
To understand the inflammatory host response at all those levels, scientists are seeking insights into the basic signaling mechanisms that go awry during infection.
“One of the big unknowns with this virus is how it induces such a strong inflammatory response in the airway,” said David Knipe, the professor of microbiology and molecular genetics in the Blavatnik Institute at HMS. “We need to understand the mechanisms of this disease because that knowledge informs everything else.”
Lab-dish experiments have shown that the virus induces certain innate signaling pathways in infected human cells, explained Knipe who leads the pathogenesis arm of the consortium with Galit Alter. However, he said, it remains unknown what immune signaling pathways are induced in tissues or in different cell types to trigger an aberrant immune response and the subsequent massive inflammation that can lead to tissue and organ damage, a hallmark of severe COVID-19. Such understanding would be critical in illuminating some of the severe complications of the disease.
Knipe, a leading authority on herpes simplex virus (HSV), has used his knowledge of HSV to study a wide range of infectious pathogens, including HIV, the West Nile virus and the SARS virus, another coronavirus that emerged in 2003. His lab developed recombinant models of HSV that express spike proteins—the molecular projections that pepper the surfaces of SARS coronavirus, MERS coronavirus and the new coronavirus, SARS-CoV-2, and enable them to invade human cells. He and his team are now adapting this model to study the immune response induced by SARS-CoV-2. Knipe is also exploring the use of herpes virus as a possible delivery vehicle—or a vector—in vaccines for COVID-19 and other diseases.
Understanding how the new coronavirus induces immune signaling inside cells—and ultimately how it does so in tissues and organs—can inform the use of existing treatments or the design of new drugs that block inflammation induced by SARS-CoV-2, Knipe said.
“To be optimally prepared for the next pandemic, we have to have a basic, in-depth understanding of microbes and how they work,” Knipe said. “The immediate priority is of course to take care of all the patients, but we need to understand the virus and how it mounts an immune response in order to develop long-term strategies for prevention and treatment.”
Another key question related to the host-pathogen interaction is whether individual immune response is related to the severity of symptoms and disease transmissibility.
One of the most striking differences between SARS-CoV-2 and its older cousins—the viruses that cause SARS and MERS—is the ability of the new virus to cause silent infections early on. COVID-19 can range from silent and barely symptomatic infections to critical illness.
Jonathan Abraham, assistant professor of microbiology in the Blavatnik Institute at HMS, studies emerging pathogens and said he would like to know whether the intensity of symptoms is in some way linked to the amount of virus in a person’s throat and airways. In other words, is there a subset of infected but minimally symptomatic people, who are unaware of their infections, unwittingly spreading the virus, emitting infectious particles with their sneezes and coughs, and leaving trails of virus behind them? This quiescent, yet highly infectious phase makes the new virus a wilier pathogen than its predecessors, allowing it to propagate more effectively and spread more widely.
“Are there people who have high levels of virus but are otherwise fine?” Abraham wondered. “This is a critical unanswered question, and the answer will become clearer in the coming weeks and months as various places across the globe ramp up their ability to diagnose infection, both serologically, with antibody testing, and by looking for viral RNA.”
Whether infection with the virus induces long-lasting immune response is another area of uncertainty. Non-peer reviewed research conducted in macaques and posted on the preprint server bioRxiv suggests that it may. The work, however, remains to be validated through peer review and then replicated in humans. If it turns out that the virus does not create long-term immune memory and that previously infected people could be reinfected upon subsequent encounters, the challenges for vaccine design would be serious.
Reinfection might occur, for example, if the virus mutates enough to evade immune recognition, essentially appearing as a new virus to the immune system upon second encounter—much as new strains of the flu virus crop up each season. Just how prone to mutations this virus is remains to be seen, experts say.
Older patients are vulnerable to worse outcomes, so another question for immunologists is elucidating precisely how the aging immune system renders older patients more susceptible to severe disease. The immune system may be weaker in older people, as well as in people with underlying health problems, and this may delay immune responses, rendering these individuals more vulnerable to severe disease.
“We are witnessing firsthand the jumping of a virus from an animal reservoir to humans, and the emergence of a new disease,” Sharpe said. “And it’s not going to be the last time this happens. Establishing the new consortium is an important first step towards building a sustainable framework to rapidly mobilize the medical and scientific expertise of our community. By developing a durable, integrated, collaborative response to SARS-CoV-2, we will be better prepared to face the next pandemic.”
THERAPEUTICS: Antivirals and antibody-based therapies
In the absence of a vaccine—which remains at least a year away—antiviral therapies would be essential in two ways. First, these treatments could be used to alleviate symptoms and avert complications among those already infected. Second, they could be given as prophylaxis to those deemed at high risk for infection or for critical illness.
Such therapeutic efforts are being pursued on two main fronts: antiviral medications and antibody-based treatments.
Antivirals
The search for antiviral medications involves the repurposing of agents already approved for other uses and the design of small-molecule antiviral drugs from scratch.
Mark Namchuk, who co-leads the therapeutics working group with Abraham, said developing antiviral drugs for COVID-19 is at its core an exercise in therapeutic translation.
“Something that's haunted the field of infectious diseases is the sheer economics of it. It's a difficult business for the for-profit sector,” said Namchuk, who is executive director of therapeutic translation at HMS and brings extensive experience in infectious diseases, inflammation biology and virology from his previous work in the pharmaceutical industry.
“It can be very difficult to make a business case to pursue this line of work. Adding a therapeutic translation piece to what we, at HMS, are doing with the consortium around SARS-CoV-2 makes a lot of sense. Most importantly, it’s 100 percent aligned with the mission of the medical school.”
This pandemic offers a striking example of how mapping the architecture of a virus and understanding its basic biology can inform the development of new treatments. Scientists already have a good idea about the structure of the virus and the part they need to go after in order to block its mischief. SARS-CoV-2 is shaped like a ball with spike proteins that form its crown-like halo. The spike protein is what the virus uses to invade human cells. To make its way inside cells, the virus attaches to the ACE2 receptor, which is found on epithelial tissue, such as the lining of the airways and lungs and, according to newly published research, in the tongue and mouth. The viral spike protein could be disabled either with a vaccine or therapeutic antibodies.
In the meantime, repurposing existing drugs may offer the easiest, most pragmatic route to rapid identification of antiviral treatments.
Two favorites right now are the Ebola drug remdesivir and the antimalarial drug chloroquine, both of which have shown some promise.
Remdesivir’s mechanism of action is especially intriguing, Namchuk said. The drug works not by inhibiting the production of viral proteins but rather by incorporating itself into the structure of the viral RNA and interfering with RNA’s ability to make more protein or replicate its genome.
“Think of it as a string of Lego blocks connected to each other to make a long chain and then inserting a block that has none of the parts that would allow the next piece to attach,”
Namchuk said. “If the virus has done all this hard work to make a string of RNA that's three, four, five hundred bases long, you incorporate one of these, well, that’s the end of it.”
Clinical trials of remdesivir have launched in several countries, including trials at Beth Israel Deaconess Medical Center and at Brigham and Women’s Hospital looking at outcomes among patients with severe and moderate COVID-19. The Brigham and Women’s trials are sponsored by Gilead Sciences, the manufacturer of remdesivir. An NIH-led study is under way at Mass General.
Besides their immediate availability, existing drugs have other appeals. Their safety profiles are relatively well-known, as are their effective dosages and possible side effects. This is especially true in the case of the antimalarial drug chloroquine, which has been around since the 1930s and has recently shown promise in a small clinical trial of patients with COVID-19. The treatment is now being tested as part of a larger clinical trial at the University of Minnesota. Elsewhere, researchers will try to determine whether giving the drug as a prophylactic treatment reduces infection rates among those at high risk for acquiring one.
But how does one screen a drug for antiviral effects outside of an actual clinical trial involving sick patients? To do so, researchers place human cells in a dish and infect them with SARS-CoV-2. As the microbe’s machinery cranks out new copies of its genome, the virus multiplies, eventually causing the infected cells to burst and die. If a drug or a chemical added to the lab dish keeps the infected cells alive, it’s a sign that the drug is halting viral replication. This is an indicator of therapeutic potential. But, Namchuk cautions, this approach currently requires level 3 biologic safety containment, which is only available in a handful of facilities. This limits the broad utility of the approach. Thus, Namchuk said, it is important to develop a testing method that doesn’t require such a high level of biosafety containment. This could be done by identifying proteins critical for viral replication and cloning them. Once isolated, these individual viral proteins can be safely tested for drug interaction in a standard laboratory benchtop outside of the confines of designated biosafety labs. In addition to screening for existing drugs, Namchuk said, this approach would enable the design of new molecules from scratch.
Another approach could be the use of virtual, or in silico, screening to comb through billions of molecules that might bind to the target protein. In silico, or computer-based, screening combined with X-ray crystallography played a critical role in the design of protease inhibitor drugs for HIV and hepatitis C. Yet, Namchuk cautioned, this approach took years to design effective molecules. So this type of drug development, while tremendously valuable, is not likely to yield insights rapidly enough to control the current pandemic, he said.
“Our current efforts are focused on what we can do now, but I think we want to keep an eye on what we can build so the next time this happens we're in a place where we can react more rapidly,” Namchuk said. “The long-term vision for this work would be to use our resources and the collaborative that we're building now to have the staying power to keep an effort on the emerging disease front even if it’s not a top-of-mind outbreak.”
In yet another example of repurposing, Megan Murray, who co-leads the epidemiology arm of the new consortium, will explore whether the BCG vaccine, currently used in many countries to vaccinate children against tuberculosis, might have protective effects against coronavirus infection.
The hypothesis is based on data suggesting that babies who received the BCG vaccine have survival advantages over babies who didn’t, an observation attributed to the vaccine’s nonspecific effects against a whole range of infections that it wasn’t designed to protect against.
“Presumably, it triggers some aspect of innate or acquired immune response, or both, which is not specific to the antigens presented in vaccine, but instead is generic,” Murray said.
She points to a study conducted in South Africa, in which adolescents were given BCG revaccinations. The results suggested that BCG revaccination also reduced other respiratory infections, most of them viral.
Murray, an infectious disease modeler whose decades-long work has focused on TB, is now designing a clinical trial to determine whether giving the BCG vaccine could offer some protection against the new coronavirus. The trial would involve vaccinating health care workers in the United States and in Peru to determine whether the vaccine—either as a first-time vaccine or revaccination—could offer protection against infection with SARS-CoV-2 in these high-risk frontline workers.
“While we wait for a vaccine specifically designed for COVID-19, we ought to explore whether BCG might help protect people who are in close contact with infected patients,” Murray said. “We think it’s really important to examine whether BCG could be of benefit early to frontline health care workers.”
Antibody-based therapy
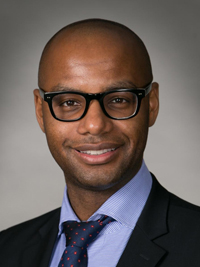
Broadly, antibody-based treatments fall in two categories: antibodies from the blood of individuals who have recovered from COVID-19 and antibodies made in the lab. Jonathan Abraham, who co-leads the therapeutic group, is part of an effort to identify such treatments. He will conduct research that involves isolating antibodies from convalescing individuals to determine how these antibodies work against SARS-CoV-2’s spike protein. The group will use imaging resources at the new Harvard Cryo-EM Center for Structural Biology to pinpoint the exact sites where their antibodies bind to the viral spike protein to disable the virus.
Antibodies against the virus (antibody-based therapies or vaccine-induced antibodies) can thwart viral access to prevent disease.
Researchers at HMS and elsewhere will harvest antibodies from patients’ memory B cells, the immune cells that form and retain memory of a microbe following infection. The antibodies will then be tested in lab dishes and in animals, including mice with humanized immune systems, to confirm they are capable of recognizing and neutralizing SARS-CoV-2.
Therapeutic antibodies could be given to infected people and used prophylactically to prevent infection in individuals at high risk for acquiring one, such as health care workers. This form of protection is known as passive immunization because it delivers ready-made antibodies instead of inducing the immune system to actively make them.
Treating sick people with immune serum from convalescing patients has been used for at least a century, but it is labor-intensive, expensive work that requires deriving and purifying blood plasma, testing it for infections and transfusing it into critically ill patients. So researchers are aiming to develop a scalable, faster alternative.
The expertise and infrastructure to develop antibody therapies on a large scale already exist, Abraham said, since antibody treatments are already being used in immune therapies for cancer and in certain autoimmune diseases. Identifying the neutralizing antibodies that can disable the novel coronavirus is what’s currently missing, Abraham said.
“The work of this consortium will be truly a case of joining forces, working together and comparing notes—whether it involves studying antibodies from infected patients or antibodies made in the lab, the idea is to move this as quickly as possible from the bedside to the bench and then back to the clinic,” Abraham said.
Designer Antibodies
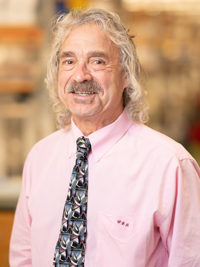
Wayne Marasco knows coronaviruses. Marasco, professor of medicine at HMS and an immunologist-oncologist with training in infectious diseases, was actively involved in the SARS outbreaks of 2003 and 2004 and in the MERS outbreak of 2012.
An inherent challenge in novel diseases is that early in the emergence of a pathogen, researchers are often unable to obtain blood samples from infected patients quickly enough. This lag curtails their ability to begin work on treatments that rely on materials harvested from the blood of infected patients, such as antibodies. To bypass that hurdle, about 20 years ago, Marasco built a library of human antibodies—27 billion of them and growing.
When the immune system encounters a pathogen—whether a microbe or a cancer cell—it produces antibodies, Y-shaped protein structures that react only to a specific antigen. The part of the antibody that changes in response to each new antigen is the pair of heavy and light chains that form the two upper arms of the Y.
Marasco has created a deep well of antibodies by separating naturally occurring light and heavy chain pairs and mixing them in countless new combinations.
“When you make these libraries, you take blood from healthy individuals and randomly mix them into a tube so you end up creating new antibody specificities that were not in the original ones,” Marasco said. “Basically, the library becomes a bottomless resource of antibody genes to highly infectious agents, to which the population had not been exposed.”
The SARS virus appeared in 2002, causing an initial outbreak in early 2003. As soon as the genetic sequence of the virus was released, Marasco and team turned to their antibody library and designed a recombinant antibody against the SARS spike protein, in effect creating the first lab-made SARS antibodies. These antibodies successfully blocked the SARS virus in lab cells, in animal models and in human blood, but failed to neutralize the SARS virus strain that emerged in the 2003-2004 season. That pathogen was markedly different because it was the product of a different evolutionary leap. The latter virus had jumped from its natural habitat—a bat—to a human, through a different intermediary host, Marasco said. That middle step altered the viral genome and resulted in a modified spike protein.
“The 2003 SARS antibodies performed poorly against the 2004 strain because the virus had evolved, so we had to find another cocktail,” Marasco said. “We learned that not all neutralizing antibodies are made the same way, and we tweaked our approach. Eventually, we found a pair of antibodies the virus could not escape from.”
What became clear, he added, is that designing an antibody therapy with a long shelf life requires designing agents that can act broadly enough to prevent the virus from escaping before it’s neutralized—preferably agents with a backup antibody that ensures neutralization if the first one fails.
“You basically have to study the virus’s evolution to anticipate viral escape and preemptively design a therapy that forces the virus into an evolutionary dead-end path from where it can’t escape,” he said.
Lack of broad neutralization can have deleterious effects beyond failing to stop the virus in its tracks. An antibody that fails to disable the virus might render the pathogen more virulent by allowing the virus to sneak into cells that it would otherwise not infect.
“Microbes evolve to stay alive,” Marasco said. “That's why they're still here. And you've got to be very careful about developing therapies that really have durability and don't cause a gain of function to make the virus more infectious.”
It’s an approach Marasco is working to replicate with the novel coronavirus.
Antibody-based therapies are not cheap, he cautioned, and they can take time to develop.
A vaccine would be the best way to contain the pandemic, he said, but there is a subset of people—anywhere between 10 million and 20 million in the United States, in his estimates—who may not be good candidates for a vaccine or would not respond to a vaccine. These include people with active cancer, immunocompromised people, pregnant people and older people whose immune systems are too weak to build vaccine-induced immunity.
“These people remain at risk and need to be treated with something—small-molecule drugs or biologic agents such as antibodies,” Marasco said. “One of the advantages of the antibody therapy is that done right, it can last a whole season.”
Marasco sees the new coronavirus as a harbinger of things to come. The fact that two coronaviruses using the exact same cell protein to invade the human body—ACE2—jumped species in a relatively short time span means it’s only a matter of time before another such viral jump occurs.
Bats are the natural reservoirs for coronaviruses. There are more than 1,400 bat species in the world—nearly a quarter of the roughly 5,400 mammalian species on Earth. Intermediary hosts can vary—civet cats for SARS coronaviruses, camels and ungulates for MERS coronavirus—so it would be prudent for people, when possible, to amend their activities around these viral sources and carriers.
“There are precursor viruses that are sitting out there,” Marasco said. “These viruses have the ability to jump species by just an accidental mutation and, given the vector and the right exposure, that could be enough. We have to be proactive and assume that there are continuous zoonotic transfers. They just don't always make it successfully. This new one did.”
Marasco said he hopes this time around, the lesson will stick.
“We have to study these pathogens in their natural reservoirs to look at the likelihood of transfer to humans,” Marasco said. “We have to ask, ‘What would it take for a zoonotic jump to occur?’ If we can do that, I think we can get a lot further in being able to recognize the potential threats and how we might mitigate against them.”
VACCINE: The ultimate quest
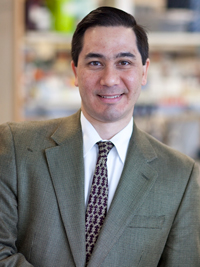
The most reliable, tried-and-true way to end a pandemic is a vaccine.
“The stunning epidemiology and clinical features of COVID-19, particularly the potential for explosive and asymptomatic spread, increase the chance that a vaccine may actually be required to end this epidemic,” said Dan H. Barouch, co-leader of the consortium’s vaccine development arm, during a recent COVID-19 grand rounds presentation.
Thus far, the behavior of SARS-CoV-2 has shown encouraging signs that a vaccine may be feasible, said Barouch, HMS professor of immunology and of medicine, director of the Center for Virology and Vaccine Research at Beth Israel Deaconess Medical Center, and steering committee member of the Ragon Institute of MGH, MIT and Harvard.
First, most people infected with SARS-CoV-2 recover. This suggests that the pathogen may induce natural immunity, which will need to be confirmed in thorough studies. Second, scientists do not know whether the virus will mutate substantially over time, but thus far it has not, which suggests that a single vaccine may be effective. Third, there is a clear target for the vaccine: the spike protein, which the virus uses to enter and infect human cells.
There are more than two dozen vaccine candidates around the world in various stages of development, including two in Barouch’s lab. These include DNA-based vaccines, RNA-based vaccines, protein-based vaccines, inactivated-virus vaccines and vector-based vaccines. The latter type uses weakened virus as a carrier to transport pieces of a pathogen to trigger an immune response.
Up until January, Barouch had never worked on coronaviruses—his previous work includes seminal studies on HIV and Zika virus, but when he and colleagues first started hearing reports of this new virus, they knew they had to get involved.
“With the experience that we had with HIV and Zika, we felt that we had an obligation to help with vaccine development for this global crisis,” Barouch said. “Since January, that feeling has only intensified.”
The SARS-CoV-2 sequence was released on Jan. 10. By Jan. 13, Barouch’s lab had ordered synthetic viral genes for two vaccine platforms. On Jan. 31, the lab entered a collaboration with the pharmaceutical company Johnson & Johnson to develop a COVID-19 vaccine using recombinant adenovirus vectors. By Feb. 6, they had immunized the first mice. The work continues in a broad range of animal models now with the hope that clinical trials in humans will begin in the fall.
While the race to a COVID-19 vaccine has moved at unprecedented speed, a widely deployable vaccine is at least a year away and perhaps longer, Barouch cautioned.
“What we need is to deploy hundreds of millions of doses of a safe and effective vaccine for the general population,” Barouch said. “This is the only relevant timeline, and so a vaccine for clinical use is still a long way off.”
Moreover, Barouch emphasized, multiple vaccines should be pursued in parallel to have multiple shots on the goal to determine the safest, most effective and most widely deployable vaccine.
LESSONS FROM TB
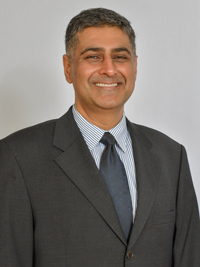
One of the fundamental mechanisms of a new disease are understood, there are different levels of translation—from basic research in the lab to diagnosis and treatment in the clinic, from clinic to practice and, ultimately, from clinical practice to population care. The final frontier of translation—and arguably the most important—is the actual delivery of screening, testing and treatment to those who need them. That is the focus of the Center for Global Health Delivery in the Department of Global Health and Social Medicine at HMS.
Lessons learned from the field of TB could be valuable in the context of the new coronavirus, according to center director Salmaan Keshavjee, professor global health and social medicine in the Blavatnik Institute at HMS
On average, TB continues to kill some 4,000 people a day worldwide, according to the World Health Organization. On March 30, COVID-19 killed 3,398 people in a single day.
The way to stop TB, Keshavjee said, is to go to communities, screen, test and treat—in place—an approach that holds lessons for the treatment of COVID-19.
“Hundreds of papers and studies in the field have shown this is the right approach, it’s just that it’s not deployed universally,” Keshavjee said. “Where it has been deployed, it has stopped transmission and largely stopped the epidemic.”
Just like TB, he added, COVID-19 should be tested for and treated in the community to the extent possible, and hospital care reserved for the very sick. Classic TB studies from the 1930s, 1940s and 1950s support that very notion.
“Early work showed that if you brought everyone in the hospital for treatment, you put them and their families at greater risk for TB, but if you treated them in the community, they fared just as well, if not better,” said Keshavjee. “Do you want to put people in hospitals if they don’t need to be there? No.”
The data from China and elsewhere shows that hospitals are hotspots of transmission. One study from China, up to 40 percent of infections may have occurred in the hospital.
“To prevent this, you want to deliver as much care as possible where people live and work,” Keshavjee said. “This epidemic requires that we think about robust community-centered screening and care in addition to what we are doing in hospitals.”
Moreover, he added, workers must be deployed where the infection is and screen in neighborhoods, test people before they get sick and give them prophylactic therapy, if and once it becomes available. Those who get sick should receive care in their homes unless they become severely ill.
“Our current approach to quarantine is not in itself a solution to contain the spread of coronavirus in communities. It’s a way to buy time to do testing and tracing and isolate carriers of the virus and their contacts so that we can stop the epidemic,” Keshavjee said. “We are doing little of that currently in the United States. Most of our activities are focused around hospitals. This is obviously necessary, but not enough.”
Any insights gleaned in the lab, Keshavjee said, need to be translated into the clinic and then, perhaps more importantly, to the communities where people live and work.
“The response to the pandemic has got to include community-wide diagnosis and the creation of 'virtual hospitals'—community based care—in the patient’s home,” Keshavjee said. “It’s the only way we are going to be able to emerge from our period of physical isolation better than we went into it.”
Community-wide diagnosis and community-based care will be easier for countries with universal health care. Epidemics have a way of exposing the vulnerabilities of health care systems. The U.S. health care system is beginning to grapple with questions such as who pays for testing and who pays for imaging and treatment. Even though some insurance companies have waived payments for testing, this is a Band-Aid approach that doesn’t solve the issue of treatment, Keshavjee said.
“What if you don’t have health insurance? And if you do have insurance, what if your emergency room copay is $150? Hopefully new legislation will address some of these issues.”
This pandemic should be an opportunity for hospitals to adopt a true hub-and-spokes model of diagnosis and care, Keshavjee said, to think about what it takes to deliver care in the communities where patients live. This approach is important for the new coronavirus, he says, but also for diabetes, heart disease, disorders of mental health and many of the other conditions that affect the lives of people worldwide.
“These are the least sexy systemic and policy issues,” Keshavjee said. “We can have all the great science and effective drugs, but if people are not getting diagnosed and treated, the human, social and economic toll on the system and, collectively, on all of us will be staggering.”